A History of Geology and Planetary Science - Part 3
From the desk of Fjordman on Mon, 2009-11-23 09:59
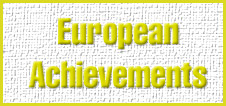
The Swedish astronomers Anders Celsius and Olof Hiorter (1696-1750) in 1741 studied the aurora borealis and noticed that it disturbed magnetic compass needles, although they could not fully explain why. While in Paris, Celsius had become acquainted with the French naturalist Pierre-Louis de Maupertuis (1698-1759) who supported Isaac Newton’s theory that the shape of the Earth swelled near the equator and slightly flattened near the poles.
To settle the matter, in 1736 the French Geodesic Mission sent one expedition to Scandinavian Lapland under Celsius and Maupertuis and another to Ecuador close to the Equator. Their measurements proved that Newton’s theory was correct. Maupertuis is also remembered for advocating the physical principle of least action and for his work on heredity before the nineteenth century theories of evolution by the naturalist Jean-Baptiste Lamarck in France and those of Charles Darwin and Alfred Russel Wallace in Britain.
At Göttingen in Germany, the physicist Wilhelm Weber (1804-1891) cooperated closely with the brilliant mathematician Carl Friedrich Gauss. The German naturalist and explorer Alexander von Humboldt in 1805 reported that magnetic intensity varied across the Earth’s surface and encouraged the establishment of an international network of magnetic observatories. By the 1830s Gauss and his younger collaborator Weber took over from Humboldt as leaders in geomagnetism. Accurate measurements were emphasized by both of them as they realized that these were crucial for developing and verifying physical laws.
During the Enlightenment it had been established that air can be electrically charged. In 1752 the Danish-Norwegian professor and bishop Erik Pontoppidan (1698-1764) suggested that the aurora borealis is an electrical phenomenon. The Scottish physicist Balfour Stewart (1828-1887), who studied terrestrial magnetism, in the 1880s proposed the existence of electrical currents in a high, conductive layer in the atmosphere to explain geomagnetic variations.
At this time the physical chemist Svante Arrhenius (1859-1927) from Sweden was developing his theory of ionic bonds, formed by the attraction between two ions with opposite charges. One example of this would be common table salt (NaCl). In the early 1800s the great English naturalist Michael Faraday had suggested that charged particles which he termed “ions” were formed by the process of electrolysis, but Arrhenius’ work led him to believe that electrolytes contain ions even when they are not exposed to electricity.
Ions are atoms that have acquired positive or negative net electric charge due to losing or gaining one or more electrons. The ionosphere is a layer of ionized air in the upper atmosphere, extending from about 80 km, where radiation from the Sun and to a lesser extent cosmic rays break apart molecules and atoms of air, leaving ions and free-floating electrons.
In 1902 the Italian physicist and radio pioneer Guglielmo Marconi successfully sent radio waves across the Atlantic Ocean. The English physicist Oliver Heaviside (1850-1925) and the American electrical engineer Arthur E. Kennelly (1861-1939) in 1902 independently predicted the existence of a conducting reflective layer that was bouncing radio waves back to the ground over vast distances in spite of the Earth’s curvature. The gifted English physicist Edward Appleton (1892-1965), who had studied under J. J. Thomson and Ernest Rutherford, in 1924 through a series of experiments proved the existence of the layer in the upper atmosphere now called the ionosphere. The ionosphere’s existence was fully established about 1930 by Appleton and Douglas R. Hartree (1897-1958) in Britain. Further studies of this layer were carried out by the English geophysicist Sydney Chapman (1888-1970).
The Norwegian physicist Kristian Birkeland (1867-1917) grew up in Kristiania (Oslo) and at the turn of the twentieth century undertook expeditions to near-Arctic regions to study aurora currents. He hypothesized that they were caused by the interaction of energetic particles from outside of the atmosphere with atoms of the upper atmosphere. He managed to experimentally reproduce the Solar System in miniature in his laboratory. He placed a magnetized sphere, a “terrella” representing the Earth, inside a vacuum chamber, aimed a beam of electrons towards it and could see that they were steered by the magnetic field to the vicinity of its magnetic poles. Birkeland’s ideas were nevertheless rejected by most scientists at the time and were only verified by satellites generations later. Even a brilliant man such as Lord Kelvin in 1892 erroneously stated that no matter passes between the Sun and the Earth.
In a drive to finance his often expensive research, Birkeland teamed up with the Norwegian industrialist Samuel Eyde (1866-1940) and invented the first industrial scale method to extract nitrogen-based fertilizers from the air. However, by the 1920s their method was no longer able to compete with the Haber-Bosch process. The German Jewish scholar >Fritz Haber<(1868-1934) invented a process, further developed by Carl Bosch (1874-1940), for mass production of nitrates, which in turn permits the mass production of fertilizers and explosives. Incidentally, Haber was also one of the developers of chemical warfare during World War I.
One large piece of the puzzle was the discovery of zones of highly energetic charged particles trapped in the Earth’s magnetic field. After the Soviet Union in 1957 launched the world’s first artificial satellite into orbit, the Sputnik 1, the United States launched its own Explorer 1 in 1958 whose Geiger counter detected a powerful radiation belt surrounding the Earth. This was the first major scientific discovery of the Space Age. The Van Allen radiation belts, named after the American space scientist James Van Allen (1914-2006), consist of two distinct parts, one inner and one outer belt, formed by somewhat different physical processes.
In 1959 the astrophysicist Thomas Gold (1920-2004) proposed the name “magnetosphere” to the highly magnetized region of space where a planet’s magnetic field dominates the plasma of the solar wind. The magnetosphere has a teardrop shape because it is compressed on the Sun side while its tail is pushed away from the Sun, similar to comet tails. Studies of comet tails by the German astronomer Ludwig Biermann (1907-1986) and others led to successful predictions of the solar wind and of the hydrogen halos around comets.
Hans Christian Ørsted in Denmark in 1820 found a connection between electrical and magnetic phenomena and opened up the study of electromagnetism. The French mathematical physicist and astronomer François Arago described the generation of magnetism by rotation in the 1820s, and his observations were expanded by Michael Faraday. As we have seen, the existence of a liquid outer core separated from a solid inner core inside the Earth was discovered in 1936 by the seismologist Inge Lehmann from Denmark. The German-born physicist Walter M. Elsasser (1904-1991) in 1946 published his theory that the Earth’s electromagnetic field is generated by an internal dynamo in the rotating, liquid outer core.
According to our best estimates, the planet Jupiter consists of almost 90% hydrogen and 10% helium by numbers of atoms, or 75/25% by mass, with additional traces of methane, water, ammonia and other chemical substances. This is believed to be close to the composition of the primordial solar nebula which existed 4.6 billion years ago. Saturn has a similar composition. The intense radiation surrounding Jupiter would be fatal to humans; its magnetosphere is immense and in volume even bigger than the Sun itself, making it arguably the largest structure in our Solar System. It is believed that Jupiter’s magnetic field is generated by an internal dynamo caused by the circulation of metallic hydrogen in that planet’s outer core.
The English geophysicists Sydney Chapman and Vincent Ferraro (1907-1974) in 1930 proposed that the Sun emits huge clouds of plasma, containing equal numbers of positive ions and electrons. It has since then been established that the Sun emits plasma at great speed at all times, not merely during magnetic storms as Chapman and Ferraro had assumed. This is the solar wind, whose existence was predicted in 1958 by the astrophysicist Eugene Parker (born 1927) at the University of Chicago in the USA against strong opposition. He developed his models when observations of comet tails still provided most of the available data. Parker’s work has greatly increased our understanding of the magnetic fields of the Earth and the Sun.
The American astronomer Fred Whipple (1906-2004) in 1950 proposed the “dirty snowball” model for comet nuclei, where comets have icy cores inside thin insulating layers of dirt. Whipple believed that jets of material ejected as a result of solar heating were the cause of minor orbital changes of comets. This model is still held to be correct. The nucleus contains a mixture of dust and water ice with elements of frozen carbon dioxide, methane and ammonia.
Comets are frozen remains of the nebula that formed our Solar System. As they approach the Sun, heat vaporizes some of the frozen materials so that the comet’s nucleus spews gas and dust particles into space. Around the nucleus, which is normally a few kilometers in diameter, comets develop a cloud of diffuse material called a coma. Comet tails are pushed away by solar radiation and the solar wind and consequently always point away from the Sun. Some scientists believe that comets originally brought to the young Earth some of the water and carbon-based molecules that make up living things, although whether substantial amounts of water were brought from comets to our oceans has been disputed based on chemical analysis.
Large sunspots may under certain conditions be seen by the unaided eye, but the modern study of them began around 1610 with the introduction of the telescope. Sunspots are strongly magnetic and appear darker because they are slightly cooler than the regions that surround them. Based on daily observation records between 1826 and 1843, the German amateur astronomer Heinrich Schwabe (1789-1875), a pharmacist living in the town of Dessau, in 1843 announced that sunspots vary in number in a cycle of roughly ten to eleven years. He was originally looking for a yet-unknown planet moving inside the orbit of Mercury. His article caught the eye of Alexander von Humboldt, who in 1851 published Schwabe’s table updated to 1850. After that many scientists became interested in the 11-year sunspot cycle. It has later been established that periods with many sunspots correspond to high solar activity.
The Swiss astronomer Rudolf Wolf (1816-1893) had studied at the universities of Zürich, Vienna and Berlin, where the German astronomer Johann Franz Encke was one of his teachers. Wolf became director of the Bern Observatory in Switzerland in 1847 and in 1848 devised the “Zürich sunspot number” to gauge the number of sunspots.
A gentleman of independent means, the English amateur astronomer Richard Carrington (1826-1875), devoted himself to the study of sunspots. Carrington found by observing their motions that the Sun rotates faster at the equator than near the poles. Another early pioneer in the study of sunspot cycles was the German astronomer Gustav Spörer (1822-1895).
The Anglo-Irish geophysicist Edward Sabine (1788-1883) in 1852 found an association between the sunspot cycle and the occurrence of large magnetic storms. On September 1, 1859, Richard Carrington in England through his telescope, which projected an 11-inch-wide image of the Sun on a screen, observed what we now know was a huge solar flare, a magnetic explosion on the Sun. Only 17 hours later this event triggered a large magnetic storm on the Earth. Just before dawn the next day, auroras occurred even in Cuba and Hawaii. Spark discharges shocked telegraph operators in several regions and set telegraph paper on fire.
Unusual solar activity can cause geomagnetic storms (disturbances in the Earth’s magnetosphere) and interrupt electromagnetic communications, for instance by affecting the ionosphere. A powerful solar flare of the strength observed by Carrington could potentially cause quite serious damage today due to our much more extensive reliance on electromagnetic equipment and communications in the twenty-first century as compared to the mid-nineteenth.
Early estimates of stellar surface temperatures made using Newton’s law of cooling gave far too high temperatures. More accurate values were obtained by using the radiation laws of the Slovenian physicist Joseph Stefan in 1879 and the German physicist Wilhelm Wien in 1896. Stefan calculated the temperature of the Sun’s surface to about 5400 °C, which was the most sensible value by date. Stefan’s Law or the Stefan-Boltzmann Law, named after Stefan and his Austrian student Ludwig Boltzmann, suggests that the amount of radiation given off by a body is proportional to the fourth power of its temperature as measured in Kelvin units.
The part of the Sun that we normally see has a temperature of more than 5500 degrees C, almost 5800 K. Temperatures in the core, where nuclear fusion occurs, reach over 15 million K. The lowest layer of the atmosphere is called the photosphere. The next zone is the chromospheres, where the temperature rises to 20,000 K. The corona, the Sun’s outer atmosphere, is remarkably hot. In the part nearest the surface the temperature is 1 million to 6 million K, but it can reach tens of millions of degrees when a flare occurs. Sunspots are cooler regions where magnetic energy builds up and is often released in solar flares and discharges of charged particles known as coronal mass ejections. These events can trigger space storms that affect the Earth. The flow of coronal gas into space is known as the solar wind. The corona is visible during total solar eclipses as a large halo of white, glowing gas, but the relative rarity of such eclipses present logistical difficulties for detailed observations.
The technical problems associated with producing an artificial eclipse to study the Sun were solved by the French solar physicist Bernard Lyot (1897-1952), an expert in optics who had studied engineering in Paris in addition to mathematics, physics and chemistry. As an astronomer, Lyot found that the lunar surface behaves like volcanic dust and that Mars has sandstorms. In 1930 he invented an instrument known as the coronagraph, a telescope equipped with an occulting disk sized in such a way as to block out the solar disk, which is more difficult than it sounds. By 1931 he was obtaining photographs of the corona. He found new spectral lines in the corona and made the first motion pictures of solar prominences.
In the 1930s, Lyot boldly inferred a coronal temperature of around 600,000 K. This claim was met with skepticism at the time. Acceptance of these very high temperatures came through the spectroscopic work of the German astrophysicist Walter Grotrian (1890-1954) and the Swedish astrophysicist Bengt Edlén (1906-1993) soon after, but an explanation for how the Sun’s upper atmosphere could be so much hotter than its surface took a long time to work out.
The Swedish physicist Hannes Alfvén (1908-1995) was one of the founders of plasma physics and magnetohydrodynamics, the study of plasmas in magnetic fields. Alfvén was born in Norrköping, Sweden. Both his parents were practicing physicians. He studied at Uppsala University and became a research physicist and professor in Stockholm. He made many discoveries in solar and space plasma physics and his work on cosmic rays led him to propose in 1937 the existence of a galactic magnetic field. The one discovery for which he is best known is the magnetohydrodynamic wave commonly called the Alfvén wave, whose existence for decades was difficult to prove. Finally in 2009, pictures taken by a team using the Swedish Solar Telescope in Spain’s Canary Islands revealed that “corkscrew” waves - Alfvén waves – were pushing heat from the Sun’s surface to its outer atmosphere, the corona.
The amount of energy the Sun puts out varies over an 11-year cycle which also governs the appearance of sunspots. While that cycle changes the total amount of solar energy reaching the Earth only by a tiny fraction, perhaps 0.1 percent, this small variation appears to be sufficient to affect our weather patterns; by how much remains a field of active research. We know that a period with very few sunspots called the Maunder Minimum, named after the English astronomer Edward Maunder (1851-1928), began around 1650, at the same time as a period of unusually cold weather called the Little Ice Age. Was this merely a coincidence?
Henrik Svensmark (born 1958) from the Center for Sun-Climate Research at the Danish National Space Center in Copenhagen, Denmark, has proposed that solar activity and cosmic rays are instrumental in determining the warming of the Earth. He builds on the work of space physicist Eigil Fiin-Christensen who with Knud Lassen Fiin in 1991 looked at solar activity over the last century and found a remarkable correlation to temperatures on our own planet.
Cosmic rays are energetic particles, most of them protons, originating from outer space. Galactic cosmic rays are subatomic particles - protons along with some heavy nuclei - accelerated to velocities approaching the speed of light by distant supernova explosions. In addition to being modulated by the Earth’s magnetic field these have to enter into the heliosphere, the protective bubble stretching far beyond the orbit of Pluto where the solar wind, the plasma of electrons and atomic nuclei constantly ejected from the Sun, dominates interstellar space. When solar activity is strong, the solar wind allows fewer external cosmic rays to reach our Solar System and our planet.
Henrik Svensmark and his colleagues carried out a landmark study of cosmic rays and clouds. They demonstrated that such rays could produce small aerosols, the basic building blocks for cloud condensation nuclei. The condensation of clouds affects the energy balance and by extension the temperature on Earth. They received support for these studies from the Danish Carlsberg Foundation, founded by the beer producer which was an early pioneer in scientific brewing. As Mr. Svensmark puts it in an online interview in the science magazine Discover:
“We live in a unique time in history, because this period has the highest solar activity we have had in 1,000 years, and maybe even in 8,000 years. And we know that changes in solar activity have made significant changes in climate. For instance, we had the little ice age about 300 years ago. You had very few sunspots between 1650 and 1715, and for example, in Sweden in 1696, it caused the harvest to go wrong. People were starving - 100,000 people died - and it was very desperate times, all coinciding with this very low solar activity. The last time we had high solar activity was during the medieval warming, which was when all of the cathedrals were built in Europe. And if you go 1,000 years back, you also had high solar activity, and that was when Rome was at its height. So I think there’s good evidence that these are significant changes that are happening naturally. If we are talking about the next century, there might be a human effect on climate change on top of that, but the natural effect from solar effect will be important.”
Far from all scientists agree that there is an intimate link between the alleged global warming going on today and cosmic rays, although the American astrophysicist Eugene Parker, the discoverer of solar wind, takes this hypothesis seriously. Nevertheless, these investigations contribute to an emerging multidisciplinary field of cosmoclimatology, the study of how “space weather” and events outside of the Earth itself may affect the climate on our planet.
According to NASA’s fine website there are at least 100 billion stars in our own Milky Way Galaxy, possibly much more than that, compared to a trillion (million times a million) or so in the huge neighboring Andromeda Galaxy. Our Solar System lies in a spiral arm about 25,000 light-years from the center of our galaxy and needs approximately 225 million years to complete one orbit of it. There are some scientists who speculate whether our position relative to the Milky Way’s center can be associated with certain geological time periods on Earth.
Scholars during the past two hundred years have vastly increased our knowledge about the chemistry of life. European chemists in the early nineteenth century made a distinction between inorganic and organic chemistry. They correctly considered the latter to be more complex, but mistakenly believed that organic substances could only be made by living creatures. This changed when the gifted German chemist Friedrich Wöhler (1800-1882), a student of the Swedish scholar Jöns Jakob Berzelius who also collaborated with the leading German chemist Justus von Liebig, in 1828 discovered that urea, an organic compound and one of the constituents of urine, could be synthesized from inorganic materials. Gradually it became clear that there is no fundamental difference between organic and inorganic chemistry apart from the fact that organic compounds are often complex. They contain carbon atoms, which have the ability to combine with other atoms in numerous different ways. The German chemist Friedrich August Kekule von Stradonitz, or August Kekulé (1829-1896), who taught at the Universities of Heidelberg, Ghent and Bonn, in 1858 established the fact that carbon has a valence (combining power) of four. This insight was of fundamental importance in the evolution of organic chemistry, which is today synonymous with carbon-based chemistry. Kekulé had the idea that carbon atoms could link up in rings as well as chains. This was independently proposed by Archibald Scott Couper (1831-1892) from Scotland as well. In 1865 described the ring structure of benzene molecules.
Carbon with atomic number six has physical properties which enable it to form millions of compounds. It has two common allotropes where its atoms are bonded together in different ways: Diamond is the hardest known naturally occurring mineral while graphite is soft and was named by Abraham Gottlob Werner from Greek for “to write” due to its use in pencils.
A more recently discovered class of carbon allotropes are fullerenes, hollow, cagelike molecules composed of at least 60 atoms of carbon. Spherical fullerenes resemble a European-style football and are called “buckyballs” after the American architect Buckminster “Bucky” Fuller (1895-1983), famous for his geodesic domes. C60 fullerene was discovered in 1985 by a team from Rice University in the United States and the University of Sussex in Britain. The English chemist Harold Kroto (born 1939) soon shared a Nobel Prize in Chemistry for the discovery with the Americans Richard Smalley (1943-2005) and Robert Curl (born 1933). Cylindrical fullerenes are known as nanotubes and are exceptionally strong.
The Russian biochemist Alexander Oparin (1894-1980) majored in plant physiology at Moscow State University and was influenced by the ideas of the English naturalist Charles Darwin. He extended Darwin’s theory of evolution backwards in time to explain how simple organic and inorganic materials might have combined into more complex compounds. In 1922 Oparin introduced the concept of a brew of organic compounds and carbon-based molecules, a “primordial soup,” as the origin of life on Earth. The English evolutionary biologist J. B. S. Haldane (1892-1964) independently proposed a closely related, though not entirely identical, hypothesis at roughly the same time. These ideas initially faced powerful opposition but have since then become accepted in their main outlines. Oparin published the book The Origin of Life and organized the first international meeting on the origin of life in Moscow in 1957.
The Dutch-born astronomer Gerard Kuiper and the American physical chemist Harold Urey (1893-1981) renewed interest in the Solar System. Kuiper discovered the carbon dioxide atmosphere of Mars and contributed to the first phase of space exploration. Urey investigated the distribution of elements in the Solar System in his book The Planets: Their Origin and Development (1952) and helped to develop the field of cosmochemistry or astrochemistry.
Harold Urey in 1921 entered the University of California to work under Gilbert Newton Lewis. He spent the following year at Niels Bohr’s Institute for Theoretical Physics in Copenhagen, Denmark. In 1931 he developed a method for distillation of liquid hydrogen which aided the discovery of deuterium, for which he received a Nobel Prize in 1934. Deuterium is a stable isotope of hydrogen where the nucleus contains one proton and one neutron. Tritium is a radioactive and very rare hydrogen isotope with two neutrons.
Urey moved to Chicago in 1945. One of his doctoral students at the University of Chicago was Stanley Miller (1930-2007), who decided to test the Oparin-Haldane theory experimentally. The famous Miller-Urey experiment from 1953 mixed water, methane, ammonia and hydrogen in a chamber to simulate the Earth’s presumed early atmosphere and used an electric discharge to simulate lightning. After just a week, organic compounds had been formed in the shape of amino acids, the basic building blocks of life as we know it.
There are those who believe that the oceans appeared as soon as two hundred million years after the Earth was formed (it was too hot before that), while others think this happened later and that primitive life forms developed soon afterward, maybe 3.8 billion to 3.5 billion years ago. The oxygen-rich atmosphere we currently enjoy, which makes complex life forms such as ourselves possible, is the result of several billion years of work by cyanobacteria, also known as blue-green algae, which use water, carbon dioxide and sunlight to produce oxygen.
Around the year 1900, several European scientists rediscovered a neglected research paper on heredity by the Bohemian monk Gregor Mendel, who had conducted breeding experiments with pea plants a few decades earlier. The American geneticist Thomas Hunt Morgan soon demonstrated that chromosomes are key factors in heredity. DNA had been isolated by the Swiss physician Friedrich Miescher already in 1869, but he didn’t grasp the importance of his find. In 1944 the Canadian-born USA-based medical researcher Oswald Avery and his co-workers more or less demonstrated that DNA itself was the unit of genetic inheritance, a fact that was further established through experiments conducted by the Americans Alfred Hershey and Martha Chase in 1952. Finally, James D. Watson and Francis Crick working at Cambridge University in England delineated the double-helix structure of DNA in 1953.
DNA, deoxyribonucleic acid, is the molecule that contains the genetic code for all currently known life forms on Earth except for some RNA-based viruses. Whether viruses constitute life forms is debatable since they have no metabolism and cannot reproduce without infecting a host cell. DNA consists of two long, twisted chains made up of nucleotides. Each nucleotide contains one base, one phosphate molecule and the sugar molecule deoxyribose. A gene is a segment of a DNA molecule that contains information for making a protein. Proteins perform the chemical reactions in our bodies and provide the body’s main building materials, forming the architecture of our cells. Amino acids are the building blocks of proteins. Chromosomes are cellular structures containing genes. Humans normally have 23 pairs of chromosomes.
During sexual reproduction the egg cell of the mother and the sperm cell of the father undergo cell division where the 46 chromosomes are divided in half and the egg and the sperm cells end up with 23 chromosomes each. The baby ends up with a complete set, half of them from each parent. In every cell in the human body there is a nucleus where genetic material is stored in genes grouped in chromosomes. Individuals suffering from the disorder known as Down’s syndrome, named after the English doctor John Langdon Down (1828-1896) who first described it in 1866, have three copies of chromosome number 21. The correct explanation for this was made by the French geneticist Jérôme Lejeune (1926-1994) in 1959.
Evolutionary biologists differ in their views of what came first, genes and then proteins or vice versa: this is the new version of the old chicken or the egg debate. Unlike double-stranded DNA, the related ribonucleic acid (RNA) usually comes as a single strand and is quite flexible. According to the website of the National Institute of General Medical Sciences, “Each year, researchers unlock new secrets about RNA. These discoveries reveal that it is truly a remarkable molecule and a multi-talented actor in heredity. Today, many scientists believe that RNA evolved on the Earth long before DNA did. Researchers hypothesize - obviously, no one was around to write this down - that RNA was a major participant in the chemical reactions that ultimately spawned the first signs of life on the planet.”
One of the most important breakthroughs in biology during the twentieth century was the realization from the 1970s and 1980s on that life on our planet is far hardier than scientists had previously suspected. Living organisms have been found in many extremely harsh and difficult environments ranging from the superheated waters of submarine volcanic vents to the ultra-dry bitter cold of the Antarctic Dry Valleys. We can encounter organisms living in boiling water or caves dripping with sulfuric acid. Most of these extremophiles are microbes.
The American research vessel Alvin, which in 1964 became the first deep-sea submersible capable of carrying a pilot and two observers to a depth of 4,000 meters, discovered black smokers in 1977 as it surveyed the Galapagos Rift in the eastern Pacific Ocean. One of the pioneers in the field of deep-water research and archaeology is Robert Ballard (born 1942), an explorer and oceanographer from the United States. Ballard is perhaps most remembered among the general public for his discovery of the wreck of the famous RMS Titanic in 1985.
Black smokers are chimneylike structures on the ocean floor made up of sulfur-bearing minerals or sulfides. Just as we can find natural hot springs in certain volcanically active regions on land, similar phenomena called deep-sea hydrothermal (hot water) vents can occur under the oceans within mid-ocean ridges, where molten rock bubbles up from the mantle to the sea floor and forms new oceanic crust. Here we can encounter unusual life forms such as tube worms and giant clams. Most notably, in this environment of perpetual darkness we can find entire ecosystems that exist totally without the aid of sunlight. They are based not on the more common photosynthesis but on chemosynthesis, by converting heat, methane and sulfur into energy and food. A few researchers such as the German chemist Günter Wächtershäuser (born 1938) have suggested that life on Earth may have begun in similar environments.
Tardigrades, sometimes known as “water bears,” are tiny water-dwelling eight-legged critters that grow to a size of about 1 millimeter. They are able to survive extreme temperatures and live from the highest mountain tops to the bottom of the oceans. In 2008 a box of water bears was launched into orbit aboard the Russian Satellite FOTON-M3 and spent ten days in containers that exposed them to the vacuum, radiation and extreme cold of space. Amazingly, some of them survived this brutal treatment and returned to Earth where they managed to lay healthy eggs. While tiny and simple, tardigrades are nevertheless multicellular organisms technically classified as animals. This was the first time that it had been demonstrated that an animal with a mouth, head, brain, legs, eyes, nerves and muscles has the ability to survive unprotected in space - an ability previously only proved for some lichens and bacteria.
A few scientists support the idea of panspermia (“all seed”), according to which life exist all over the universe, or the more moderate concept of exogenesis (“outside origin”) where life on Earth originated elsewhere, maybe in the form of extraterrestrial microbes brought here with meteorites. These hypotheses are highly controversial and remain the view of a very small minority of scholars, but the fact that a few microscopic terrestrial organisms can survive in space is certainly interesting. Since life on our planet is hardier than we expected this increases the likelihood that it can exist elsewhere, too. This is especially intriguing now that we finally have the technological capability to explore other bodies in our Solar System.
The polymath Mikhail Lomonosov (1711-1765) was a pioneer of modern science in the Russian Empire. Born in a small village, his family were nominally peasants but still enjoyed a degree of freedom not known to serfs in Central Russia. He concealed his identity, as peasants could not attain the prestigious Academy in Moscow, and pretended to be the son of a priest to gain admission. He soon impressed his teachers with his intelligence. In 1735 he was selected to the new Imperial Academy of Sciences in St. Petersburg. Lomonosov studied for several years in Western Europe and picked up a German wife. In 1748 he opened the first modern chemical laboratory in Russia. He also promoted Russian history and language.
According to the book Venus in Transit by Eli Maor, “It was during the 1761 transit that Lomonosov, observing from his home in St. Petersburg, saw a faint, luminous ring around Venus’s black image just as it entered the sun’s face; the sight was repeated at the moment of exit. He immediately interpreted this as due to an atmosphere around Venus, and he predicted that it might even be thicker than Earth’s. Lomonosov reported his finding in a paper which, like most of his written work, was only published many years after his death in 1765. But it was not until 1910, one hundred and fifty years after the transit, that his paper appeared in German translation and became known in the West. Up until then the discovery of Venus’s atmosphere had been credited to William Herschel.”
The Venera 7 probe from the Soviet Union in 1970 became the first space probe to transmit data from the surface of Venus. The Earth’s atmosphere is by volume composed of roughly 78% nitrogen gas (N2), 21% oxygen gas (O2), 0.9% argon (Ar), some water vapor (the gas phase of water, H2O), almost 0.04% carbon dioxide (CO2) and small amounts of a number of other gases such as methane (CH4). While the three latter gases constitute a tiny part of the atmosphere they trap heat from the Sun and warm the Earth through the greenhouse effect.
The mass of the atmosphere of Venus consist of 96.5% carbon dioxide and the planet is surrounded by thick clouds of sulfuric acid. The dense atmosphere produces a run-away greenhouse effect and the temperature of Venus’ surface is more than 460 degrees Celsius, hot enough to melt lead. It is highly unlikely whether life as we can conceive of it can exist in such an inhospitable environment, except possibly in the cooler upper atmosphere. The Martian atmosphere, too, is dominated by carbon dioxide at 95.3 percent plus some additional nitrogen, argon and water vapor, but at the surface the atmospheric pressure is typically 0.7 percent that of the Earth’s surface. In contrast, atmospheric pressure at the surface of Venus is about 92 times that of the Earth and by extension roughly ten thousand times that of Mars.
The astronomer Giovanni Schiaparelli (1835-1910) from the winy Piedmont region of northwestern Italy explained regular meteor showers as the result of the dissolution of comets and proved it for the Perseids, thereby forging a link between comets and certain meteors. He studied Mars and named its “seas” and “continents.” According to the book The Planet Mars: A History of Observation and Discovery by William Sheehan, “In Italian, canali can mean either ‘channels’ or ‘canals.’ It is clear that Schiaparelli had completely natural features in mind---indeed, he often used the word fiume (river) as a synonym. Strictly speaking, the term channel would have been preferable, but instead it was canal, with all its connotations of artificial waterways, that was adopted in English, with far-reaching consequences.”
Schiaparelli’s alleged observations of Martian canals stimulated the American businessman and astronomer Percival Lowell (1855-1916) to found his observatory in the 1890s and search for intelligent life on Mars. He also predicted the existence of a planet beyond the orbit of Neptune. The young American astronomer Clyde Tombaugh (1906-1997) discovered Pluto in 1930 while working at the Lowell Observatory in the USA. He used photographic plates, which were used in astronomy and particle physics long after they had gone out of popular use, although astronomers like many others later switched to digital cameras. In Roman mythology Pluto, the equivalent of the Greek deity Hades and his abode of the same name, was the god of the dark underworld. “PL” also happens to be the initials of Percival Lowell.
Our seasons are caused by the Earth being tilted on its axis by 23.5 degrees. This axial tilt is not constant but varies very slowly from 22.1 to 24.5 degrees in a cycle of 41,000 years. In June the Northern Hemisphere faces the Sun and receives more direct sunlight, which means that there is summer in the north and winter in the Southern Hemisphere. The exact opposite is the case six months later when the Earth is on the other side of its orbit and is tilted the other way vis-à-vis the Sun. The seasonal daylight differences and the gradual lengthening or shortening of days are subtle in the tropics, but the regions next to the poles will be in total darkness by mid-winter and in turn receive 24 hours of nonstop sunlight (the “midnight Sun”) in the middle of the summer, close to winter solstice and summer solstice, respectively.
Mars has an axial tilt of 25.2 degrees and has seasons just like the Earth, only longer ones since the Martian year - its orbital period around the Sun - is longer with 687 Earth days. One crucial difference is that while the Earth’s orbit is nearly circular, Mars has a significantly more elliptical orbit with a more pronounced orbital eccentricity. This means that the difference between the point when it is closest to the Sun, the perihelion, and the point when it is furthest away from the Sun, the aphelion, is small and climatically insignificant for our own planet but significant in the case of Mars. While the Martian atmosphere is thin it is still dense enough to support a weather system. Huge dust storms can cover almost the entire planet and are often strongest at perihelion when the Sun heats its atmosphere the most.
Mars is named for the ancient Roman god of war. Its reddish color, similar to that of rust (iron oxide), comes from iron-rich minerals in its soil. Large amounts of water probably once flowed on its surface, which contains many channels, gullies and huge valleys. The planet’s seasonal polar caps consist of a mixture of solid carbon dioxide (“dry ice”) and water ice.
Since there are currently no seas on Mars there is no “sea level” to measure from, but by any standard the massive shield volcano Olympus Mons is the largest known mountain in the entire Solar System. Standing about 25 kilometers higher than its surrounding landscape it is almost three times higher than Mount Everest. However, the force of gravity at the Martian surface is only about 38 percent that of the Earth. A mountain of similar size probably wouldn’t have survived on our planet as it would have been crushed under its own weight.
While it remains a possibility that plate tectonics once existed on Mars, astrogeologists believe that plate tectonics processes are no longer active there. Olympus Mons is thought to be fixed over a deep hotspot, which has allowed repeated eruptions to build it to its present height. On the Earth, hotspots remain stationary while crustal plates are moving above them. It is virtually certain that our hot sister planet Venus is still volcanically active. It is distinctly possible that cooler Mars is so as well, but we currently possess no direct evidence of this.
Mars has no global magnetic field, which means that its core is probably entirely solid. The Earth’s magnetic field creates a protective bubble against the solar wind and harmful rays from space. Since Mars lacks this and has a thinner atmosphere, the planet’s surface is much more exposed to potentially harmful radiation. The average temperature is about -60 degrees Celsius, but varies considerably seasonally and regionally from a comfortable 20 degrees plus near the equator at summer to a staggering minus 130 degrees Celsius near the poles during the winter. Because of these factors, if evidence of past or present microbial life is ever found on Mars, many astrobiologists suspect that it will be located just below the surface. There, microbes might be more shielded from cosmic radiation, and heat from the still hot Martian core makes it more likely that non-trivial amounts of water could still exist in liquid form.
Joan Oró Florensa (1923-2004) was a Catalan biochemist who graduated from Barcelona University in 1947 and emigrated from Spain to the USA in 1952. Following the Miller-Urey experiment from 1953, Joan Oró in 1959 demonstrated in a related experiment that adenine, one of the components of DNA, formed in abundance in his “primordial soup.” Oró was one of the first scientists to suggest the possibility that comets could have acted as carriers of organic molecules to the Earth’s early biosphere. From the 1960s he worked with NASA, including the Viking missions to Mars in 1976. While the results were inconclusive back then, it is possible that their equipment was not sensitive enough to detect life forms even if present.
In 2003-2004, American space scientists as well as data from the Mars Express Orbiter by the European Space Agency (ESA) found evidence of methane in the Martian atmosphere. There could be an active source of methane production on the planet. This is not by itself proof of the presence of life as methane can be produced through both biological and non-biological processes, but the discovery is definitely encouraging. Obviously, if we do find extraterrestrial life on Mars or elsewhere it is not at all certain that it will be carbon-based and DNA-based as life is on our own planet. Theoretically speaking, extraterrestrial life could be so different from the life forms we are familiar with that we would find it hard to recognize it as life at all.
From the Age of Exploration until the twentieth century, European explorers and eventually scholars reached almost all corners of the planet, including the polar region. Vitus Bering (1681-1741), a Danish navigator in the service of the Russian Navy, was the first known European to see Alaska. The Bering Strait, which separates Siberia and the Asian continent from North America, is named after him. Across this strait there was a land bridge during the last Ice Age. It is likely that nomadic hunters from Siberia entered Alaska and the Americas from here. There is archaeological evidence of the widespread presence of the Clovis culture in North and South America before 10,000 BC, but it is remains possible that there were several different waves of settlement and that the first one began even earlier than this. The expeditions of the American explorers Frederick Cook (1865-1940) and Robert Peary (1856-1920) to the North Pole region and those of the Norwegian explorer Roald Amundsen (1872-1928) and the Anglo-Irish explorer Ernest Shackleton (1874-1922) to Antarctica, while greatly fascinating, were not noted primarily for their scientific interest; they were more about glory and adventure in addition to the possibility of discovering new and potentially important sea lanes. Modern polar science began with the first International Polar Year in 1882. This was the brainchild of the officer Carl Weyprecht (1838-1881) of the Austro-Hungarian navy, who had discovered Franz Josef Land in 1874 while searching for the Northeast Passage.
Expeditions to Greenland by the Norwegian explorer and scientist Fridtjof Nansen (1861-1930) in the 1890s and by Alfred Wegener from Germany in the early 1900s surveyed the glaciers and ice sheets there. The same was the case with the British Royal Naval officer Robert Falcon Scott’s (1868-1912) Antarctic expedition of 1901-1904. In recent decades, ice sheets have gained international attention for preserving some of the finest records of climate change over the last hundred thousand years or more. Glaciology, the study of ice, ice formations and glaciers, is also of major importance to planetary scientists.
Next to Mars, the most promising candidates for primitive life in our Solar System might not be the planets but rather some of their moons. The study of ice on bodies such as Jupiter’s moons Europa, Callisto and Ganymede as well as Saturn’s icy satellites Enceladus with its large water vapor geysers and especially Titan with its dense atmosphere and surface liquid in the form of hydrocarbon lakes is an emerging scientific field. Ganymede is the largest satellite in the Solar System. Like Titan it is larger in diameter than the innermost planet Mercury but has less mass. Mercury is a dense object. Europa may harbor a liquid water ocean and is therefore a priority target for future space probes. The same could be true of Callisto. The fourth of Jupiter’s large Galilean moons, Io, is the most volcanically active body in the Solar System, with volcanoes spewing out sulfur and sulfur dioxide to a height of hundreds of kilometers. The heat is caused by massive tidal forces generated by Jupiter and other moons. Volcanism exists on several bodies, though not necessarily in the form of molten rock (lava) as we can see here on Earth. The path-breaking American Voyager 2 spacecraft in 1989 observed cryovolcanoes (ice volcanoes) on Triton, the largest moon of the planet Neptune. The temperature at the surface of Triton is only 34.5 K (-235 C), at least as cold as Pluto. In this extreme cold methane, nitrogen and carbon dioxide all freeze solid. The geysers Voyager 2 observed on Triton are probably nitrogen geysers driven by seasonal heating by the Sun.
A recent advance of tremendous importance is the discovery of the first extrasolar planets, planets orbiting other stars. Hundreds of these have been found during the first generation after 1990 alone. This has led to the establishment of a new branch within planetary science dubbed exoplanetology or exoplanet science. Most of the planets discovered so far have been gas giants detected through indirect means by observing the effects they have on the stars they orbit, but methods are rapidly improving and more Earth-like planets have been identified. It is highly unlikely that we in the foreseeable future, if ever, will have the technological capability to send robotic probes to explore extrasolar planets, let alone manned missions. Nevertheless, by studying them from a distance we can learn a great deal about planet formation and about how common Earth-like planets are in our galaxy and in the universe.
Unlike most elements lighter than iron the alkali metal lithium with atomic number three is not easily produced in stars. According to current theories, most of it was probably created after the Big Bang. Yet astronomers see a wide range of different lithium levels in Sun-like stars. With the European Southern Observatory’s HARPS spectrograph survey of hundreds of stars, astronomer Garik Israelian of Spain's Instituto de Astrofisica de Canarias in Tenerife and his colleagues found that those that had an orbiting planetary system had lithium levels similar to the Sun’s while those that did not had higher levels. If this insight is correct it might suggest an easier way to look for undiscovered planetary systems around other stars.