The Experimental Method and the Rise of Modern Science
From the desk of Fjordman on Fri, 2010-01-22 07:32
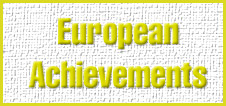
We should be careful about projecting a too modern understanding of “science” onto the activities of ancient scholars. As the eminent historian Edward Grant reminds us, “Science in the ancient world was a tenuous and ephemeral matter. Most people were indifferent to it, and its impact was meager. It was a very small number of Greek thinkers who laid the foundations for what would eventually become modern science. Of that small number, a few were especially brilliant and contributed monumentally to the advancement of science.”
This is not said to dismiss them or downplay the significance of what they did. On the contrary, we should be all the more impressed by their achievements given how few they were and the limited resources they had at their disposal. Nevertheless, while the ancient Greek contribution was substantial and important it was only one of the major components of what would become modern science when this was finally created in early modern Europe.
Hipparchus, with his star catalog from the second century BC, was the first to introduce a system for measuring the brightness of stars with six levels of magnitude and the brightest ones in class 1. Today’s system essentially follows the same logic. Some stars are so bright that they even have negative magnitudes, for instance Sirius with a visual apparent magnitude of −1.46. Apparent magnitudes do not take into account the distance of the star in question from the Earth. Absolute magnitude is when you directly measure a star’s luminosity.
Robert Grosseteste (ca. 1170-1253) was a talented English scholastic philosopher, Bishop of Lincoln and commentator on the works of Aristotle. Grosseteste introduced Latin translations of some Greek and Arabic philosophical and scientific writings and around 1230 he was probably teaching at the then very young University of Oxford. He composed a number of short works regarding optics and experimented with mirrors and lenses. He proposed that a theory can only be validated by testing its consequences with physical experimentation.
This deviation from traditional Aristotelian philosophy has earned him, with some justification, the reputation of being a pre-modern supporter of the scientific method. Grosseteste’s student Roger Bacon (ca. 1220-1292) doesn’t deserve the label as the “founder of experimental science” that is sometimes bestowed upon him, but he was an influential advocate of gathering empirical evidence in the sciences and he practiced what he preached.
Another pre-modern pioneer from the thirteenth century was the French natural philosopher Peter Peregrinus of Maricourt, who used an unsophisticated version of the experimental method in his work Epistola de magnete from 1269. This was the first extant written account of the polarity of magnets and proved exceedingly popular in the generations that followed.
One of those who quoted it was the English scholar and theologian Thomas Bradwardine (ca. 1290-1349), who was educated at Merton College, Oxford. Bradwardine was a noted mathematician as well as theologian and died in London of the plague during the Black Death after having served briefly as Archbishop of Canterbury. Later in the fourteenth century the author Geoffrey Chaucer would rank him next to Saint Augustine and Boethius in importance.
The English Franciscan friar William of Ockham (ca. 1287-1347), sometimes spelled Occam, was among the most prominent philosopher-theologians of the High Middle Ages, next to John Duns Scotus (ca. 1266-1308) and a handful of others. He studied theology at the University of Oxford before 1320 and was called to the Papal court at Avignon, France in 1324 to answer charges of heresy. In 1328 he moved to Munich where he remained until his death. Around 1320 he developed what has become known as Ockham’s Razor. He was not the first person to mention the principle of parsimony but he championed it so systematically that it has been named after him. Briefly formulated it says “Don’t multiply entities beyond necessity,” in other words use as few assumptions as possible and prefer the simplest explanation that fits the available data. This remains a crucial principle in scientific logic.
Aristotle’s ideas were not always treated uncritically in ancient times. Strato of Lampsacus (ca. 335-269 BC), the third head of the Lyceum after Aristotle himself and his successor Theophrastus, criticized Aristotle’s lack of attention to the speeding up and slowing down of bodies as they begin or end their motion. The sixth-century Eastern Roman natural philosopher John Philoponus added to this debate regarding Aristotle’s theories of motion.
Galileo’s dynamics and kinematics of motion drew substantially from fourteenth-century developments at the Universities of Oxford and Paris. The great French mathematician Nicole Oresme did notable work on projectile motion and was critical of some of Aristotle’s views on the subject. He was taught by the highly influential French priest and philosopher Jean Buridan (ca. 1300-1358) at the University of Paris in the early 1340s. They followed in the footsteps of John Philoponus, whose impetus theory was reaffirmed by Buridan and Oresme.
In the eyes of David C. Lindberg, a few breakthroughs often attributed to the seventeenth century had ancient or medieval roots. In some cases we can find that “revolutionary achievements in many disciplines were built on medieval foundations and out of resources provided by the classical tradition. Revolution does not demand total rupture with the past.”
The mechanical philosophy, a centerpiece of the Scientific Revolution, was aided by a revival of Epicurean atomism from ancient Greece which had been preserved through the writings of Roman authors, passed down with the Classical tradition and Christianized. It was employed in the seventeenth century by men such as Pierre Gassendi, Robert Boyle and Isaac Newton.
Another important branch of science where there was some continuity was optics. Mr. Lindberg has successfully demonstrated that while Kepler in the early 1600s formulated the first modern optical theory, he built it on the foundations of optical studies dating back to Ptolemy and developed further by medieval scholars such as Alhazen, Kamal al-Din, Theodoric of Freiberg, Robert Grosseteste and Roger Bacon. All of these men had at least some rudimentary understanding of the experimental method, even Ptolemy in the Roman era, yet this did not then evolve into a methodical use of it or become widely adopted by others.
It is almost certainly possible to find isolated cases where Chinese, Indian or other thinkers employed unsophisticated versions of the scientific method; what was entirely novel in seventeenth century Europe was a sustained program of experimentation successfully promoted by influential men such as the English philosopher and writer Francis Bacon. That was new and revolutionary. David C. Lindberg writes in The Beginnings of Western Science:
“Peter Peregrinus of Maricourt manipulated magnets in order to gain an understanding of their properties and behavior – discoveries that anticipated many of those that would subsequently be made in the seventeenth century by William Gilbert, often identified as one of the founders of experimental science. And who could deny the status of experimental scientist to the thirteenth-century Franciscan friar Paul of Taranto, who initiated an alchemical tradition characterized methodologically by laboratory manipulation of substances in the attempt to discover the pathway to transmutation? ... If all of this is true, what credit is left for Francis Bacon (1561-1626), popularly celebrated as the founder (or a founder) of experimental science? This Bacon (no descendent of Roger) argued, in books filled with references to empiricism and experiment, for the experimental interrogation of nature. However, what he and the Baconian tradition of the seventeenth century gave us was not a new method of experiment, but a new rhetoric of experiment, coupled with full exploitation of the possibilities of experiment in programs of scientific investigation.”
Edward Grant, on the other hand, chooses to emphasize that “Science in the late ancient and medieval periods, was, however, radically different from modern science. Although some interesting experiments were carried out, they were relatively rare occurrences and certainly did not constitute a recognized aspect of scientific activity. Few claims were tested objectively. The experimental method did not yet exist. The mathematical sciences, however, were presented with the same kind of vigor as a modern treatise in mathematical physics.”
In mathematics it is not at all difficult to find certain elements of continuity. Euclid’s Elements from around 300 BC incorporated even older Greek, Egyptian and Babylonian geometry and has been studied by Western students of mathematics until the present day.
Before 200 BC, the Conics by the great Hellenistic Greek geometer Apollonius of Perga introduced ideas and terminology that influenced later scholars from Ptolemy to Newton. As John North states, “He did for the geometry of conic sections (parabola, hyperbola, line-pair, circle, and ellipse) what Euclid had done for elementary geometry. He set out his own work, and much of that done by his predecessors, in a strikingly logical way. He also showed how to generate the curves using methods strongly reminiscent of those used in modern algebraic geometry. Those methods were to prove enormously important to astronomy, in the century of Kepler, Newton, and Halley – who studied Apollonius’s text closely.”
According to author Stephen Gaukroger in his book The Emergence of a Scientific Culture it is wrong to compare the totally unprecedented efforts of the Scientific Revolution in early modern Europe, which is the Scientific Revolution, to the more limited creative periods in other civilizations and time periods. The difference is not merely one in degree, but in kind:
“Scientific developments in the classical and Hellenistic worlds, China, the medieval Islamic world, and medieval Paris and Oxford, share a distinctive feature. They each exhibit a pattern of slow, irregular, intermittent growth, alternating with substantial periods of stagnation, in which interest shifts to political, economic, technological, moral, or other questions. Science is just one of a number of activities in the culture, and attention devoted to it changes in the same way attention devoted to the other features may change, with the result that there is competition for intellectual resources within an overall balance of interests in the culture. The ‘Scientific Revolution’ of the early-modern West breaks with the boom/bust pattern of all other scientific cultures, and what emerges is the uninterrupted and cumulative growth that constitutes the general rule for scientific development in the West since that time….This form of scientific development is exceptional and anomalous. The question is, then, not why the Scientific Revolution didn’t occur in any of the other cases of rich, innovative scientific cultures, but why it occurred in the West.”
De Magnete (“On the Magnet”) from the year 1600, written by the English physician and natural philosopher William Gilbert (1544-1603), became the standard work on electrical and magnetic phenomena at a time when Western European nations were engaged in long sea voyages and needed knowledge about the workings of the magnetic compass. Gilbert likened the polarity of the magnet to the polarity of the Earth itself. His work included descriptions of some of his own experiments as well as data that had been obtained by others. Galileo was very interested in Gilbert’s magnetic researches as well as his experimental methodology.
The Italian natural philosopher, astronomer and instrument maker Galileo Galilei (1564-1642) was educated at home and with monks before he enrolled at the university in his native city of Pisa in 1581. He left without a degree, but continued to do independent research. He had to make a living by teaching and tried to generate additional income by inventing various devices, among them an early thermometer which was only a limited success. He was appointed professor of mathematics at Pisa in 1589. From 1592 to 1610 he held a similar position at Padua. During these years he studied falling bodies but did not publish his findings at that time. Had Galileo died in the early 1600s he would have been virtually unknown today.
Whether he literally dropped weights from the Leaning Tower in Pisa, as legend has it, is debatable, but the Flemish scholar Simon Stevin had conducted similar experiments in 1586. His results were published and may have been known to Galileo. Galileo demonstrated that heavy and light objects, aside from the effects of air resistance, fall at the same rate. Aristotle claimed that heavy objects fall more rapidly than lighter ones. Galileo showed that a falling object increases its speed in a steady fashion, or undergoes uniform acceleration. At sea level on our planet this gravitational acceleration roughly equals 9.8 m/s² for all objects. He established the principle of inertia whereby a moving object in the absence of external forces will continue to move with undiminished speed. Aristotle claimed that it would slow down.
What set Galileo apart from most scholars of ancient and medieval times and clearly separated him from Copernicus a few decades earlier was that he embraced the philosophy of the “experiment” as a controlled situation in which specific phenomena could be produced and studied at will. This was, as we have seen, not necessarily an entirely new idea, but it was taken up with a new enthusiasm in the late 1500s and early 1600s. A greater emphasis on empiricism and experiment could be detected from Italy via the Low Countries to England.
William Harvey (1578-1657), a physician from Elizabethan England who studied at the University of Padua after 1598 under Galileo’s anatomist colleague Hieronymus Fabricius (1537-1619), was the first person to correctly describe the circulation of the blood. Jan Baptist van Helmont (1579-1644), a Brussels-born Flemish physician, in the early 1600s carried out a famous experiment by growing a tree in a pot for five years. Through careful measurements he deduced that its increased mass came from the water, which was partly correct; it also came from atmospheric carbon dioxide and sunlight through the process of photosynthesis.
After 1609 Galileo became heavily involved in astronomy with the newly invented telescope. The sensational observations he published in 1610, including his discovery of the four largest moons of Jupiter, brought him instant fame. His Dialogue Concerning the Two Chief World Systems from 1632 with its favorable view of the Copernican system got him into the famous controversy with the Roman Inquisition. While under house arrest near Florence he completed his final masterwork, Two New Sciences (1638), which was published in Leiden in the Netherlands. Here he summarized investigations he had conducted decades earlier regarding the strength of materials and the motion of objects and anticipated Newton’s laws of motion.
Galileo was a firm believer in the application of mathematics to physics, not only for heavenly objects such as planets but for the study of motion of everyday objects here on Earth. It was his instinct for mathematical modeling of physical phenomena combined with his groundbreaking experiments and systematic use of the scientific method that earned him the well-deserved reputation he now enjoys for being a, if not the, founder of modern physics.
There is an immense gulf between societies in which science was the domain of a handful of wise men and our society with thousands of specialists seeking to contribute to a coherent understanding of all natural phenomena. Modern Europe contributed a unique institutional invention in world history: a large, organized body of scholars seeking explanations of all natural phenomena by a common method based on observation, experiment and reason. Europeans were aided in this by better scientific instruments, physical as well as mathematical ones. Authors Nathan Rosenberg and L.E. Birdzell Jr. explain in How The West Grew Rich:
“What made the difference to the creation of organized science was that the experimental method was adopted by a number of researchers, and their common method united them in a community of working scientists. Post-Galilean natural science could specialize and departmentalize into physics, astronomy, chemistry, geology, biology, and a host of narrower specialties because all of them shared a common method of determining scientific truth. A geologist or biologist could use the teachings of physics or chemistry in geological or biological research without feeling the need (or even the possibility) of checking their validity. The general acceptance of the experimental method made it possible for hundreds and even thousands of specialists to build the results of their individual research into a single store of information, usable across all sciences. The introduction of the printing press greatly speeded the cumulation of this body of knowledge….Thus the West, alone among the societies of which we have knowledge, succeeded in getting a large number of scientists, specialized by different disciplines, to cooperate in creating an immense body of tested and organized knowledge whose reliability could be accepted by all scientists.”
In his 1996 book The Scientific Revolution, professor of sociology Steven Shapin questions whether there was such a phenomenon at all. Most historians hold that a new pattern did emerge during the seventeenth century. Scholars questioned and occasionally ridiculed some of the ancient wisdom that had been inherited from previous ages. Many “new men” were critical of the established universities, which were seen as having invested too much faith in past authority, particularly in Aristotle. This was in sharp contrast to the Renaissance, which was preoccupied with Antiquity and had relished ancient knowledge; the older, the better.
A new pattern of organized science based on experiment emerged, less tied to Aristotelian natural philosophy and open for reports from non-academics. This development found its institutional basis in the scientific societies. During the 1660s the Royal Society of London for the Improvement of Natural Knowledge, the longest continuously enduring scientific society in the world often known simply as the Royal Society, was created. Similar set-ups followed from Berlin to St. Petersburg. European scholars developed networks and created increasingly sophisticated descriptions of their experiments for others to trust in or duplicate and verify for themselves. Here we find the seeds of what would gradually evolve into scientific journals.
The universities eventually caught up with this, too. The influential Dutch professors Willem Gravesande (1688-1742) and Pieter van Musschenbroek (1692-1761), the inventor of the Leiden jar, at Leiden University gave the first sustained university courses in natural philosophy illustrated with experiments. By 1750 many professors were introducing experiments into their courses. Charles-Augustin de Coulomb used long, thin magnetic needles with well-defines poles to establish the law of magnetic force. Antoine Lavoisier used his self-made apparatus for experiments on gases, respiration and heat in the 1770s and 80s.
The word “scientist” was not seriously used much before 1840, and not widely used until the twentieth century. “Man of science,” “thinker” or “scholar” should be considered preferred labels for those who worked with natural philosophy and what we call science prior to this. By the mid-eighteenth century, probably no more than 300 people in the world could be classified as scientists. By the year 1800 there were perhaps a thousand, by the mid-nineteenth century 10,000 and by 1900 maybe 100,000. The overwhelming majority of these were still Europeans or people of European origins. The European population itself grew rapidly at the same time, but the percentage of scientists grew even faster. Science during this period finally made the transition from being a gentleman’s hobby to being a well-populated profession.